The microbial desalination cell (MDC) is a technology that allows desalinating water through bio-electrochemical reactions [1]. An MDC is composed of three main parts including anode, water and cathode chambers (separated by anion exchange and cation exchange membranes, respectively) (figure 1.a). MDCs work according to microbial fuel cell fundamentals. The anode chamber is inoculated with a mixed culture of bacteria (e.g., anaerobic sludge) to degrade the substrate in order to produce green electricity. Current generation in anode leads to protons release through redox reaction pathways. However, produced protons are blocked by the anions exchange membrane, and the negatively charged species migrate to anode instead. Positively charged species leave the water chamber towards the cathode side at the same time. As a result, desalinated water is generated. Indeed, MDC technology is an environmental-friendly approach to desalinate water, produce bio-energy and treat waste water simultaneously. Ion addition to the anode and cathode chambers increases the conductivity of wastewater streams and increases electricity generation. On the other hand, increased salinity detrimentally affects effluent treated water quality and plays an inhibitory role to biological waste water treatment in the anode [2-3].
Microbial capacitive desalination cells (MCDC) are a suitable alternative to overcome salt transfer to waste water and pH fluctuation problems in MDCs (figure 1.b). They promote CDI efficiency as well [4]. A capacitive system is installed in the middle chamber of MDC and two cation exchange membranes are utilized instead of the anion and cation exchange membranes. Microorganisms generate an electrical field to drive the electrochemical ion adsorption in MCDC. Two membrane assemblies including current collector, activated carbon cloth and cation exchange membrane is installed and connected to the anode and cathode (figure 1). The ions are stored in the electric double layer formed on the activated carbon/solution interface instead of the treated waste water [5]. This assembly can be recovered through electrical filed elimination during the discharge of activated carbon. Another privilege of MCDI over MDC is to obviate unfavorable pH gradients due to blocking of protons in the anodic side. In fact, MCDC facilitates the protons movement in entire cell using cation exchange membranes [4-5].
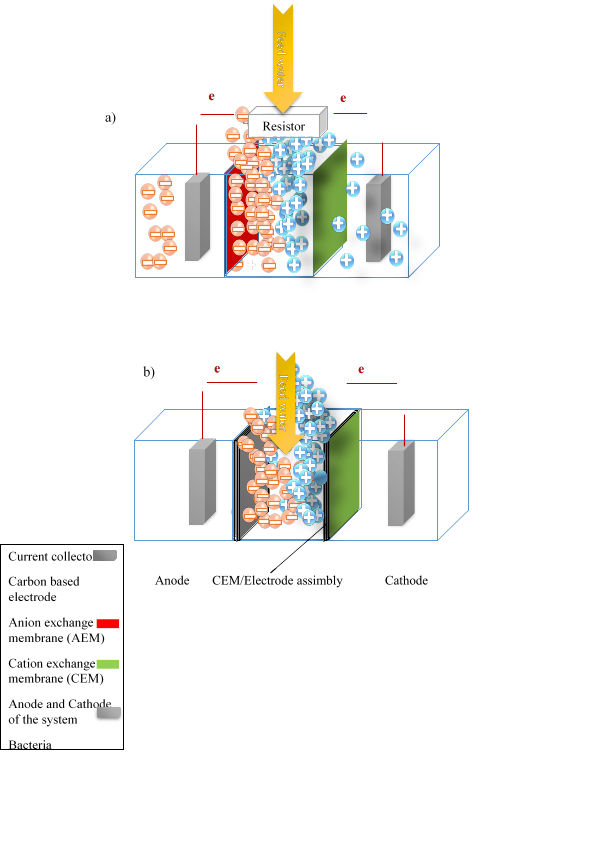
Reverse osmosis (RO), as a dominant desalination process, still needs cost optimization [6]. Recently, other novel membrane-based desalination technologies have been considered as RO pretreatment alternatives to reduce costs. Reverse electro-dialysis and forward osmosis are interesting pretreatment options. Recall that membrane technologies have poor removal performance for organic hydrocarbons [7-9]. Consequently, another complimentary step is taken to eliminate organic compounds [8-9]. Therefore, technologies that remove both organic matter and salts, are preferred. Reduction of energy consumption is another vital parameter to invest on. Undoubtedly, capacitive deionization is a potential candidate to meet the above-mentioned requirements properly. Architecture diversity and flexible design of CDI makes it a unique system to be coupled with other desalination, energy storage and biological treatment technologies. In addition to energy costs, the cost of membrane and its maintenance may be attenuated in these systems. Biological and organic matter fouling along with the scaling problem are controversial issues in a long-term run [10-11]. It seems CDI faces fewer problems such as fouling and scaling with respect to membrane technologies. Long term research on CDI systems may provide insight to these controversial issues. To accelerate commercialization of CDI systems, special focus on the following items would be beneficial: Development of new cost-effective electrode materials and long-term performance analysis. In order to reduce the energy cost, an alternative approach has been considered. Green energy supplies from new sources including wastewater (e.g., MCDC), water salinity difference in capacitive mixing systems, and gas phase CO2 concentration difference have been recommended [12]. Novel integrated structure designs facilitate desalination as well as energy harvesting from available unused resources. Lastly, it is highly recommended to focus on real brackish water and industrial effluents to extract ions from a real complex mixture in future research. Each water source has its own characteristics and should be analyzed carefully before any study. Although it is not possible to generalize the result of a specific application to other case studies, comprehensive assessments can be achieved according to similarities.
References
[1]Xiaoxincao, † X iahuang , Pengliang , Kangxiao, Yingjunzhou, Xiaoyuanzhang ,and Bruce.E. Logan. A New Method for Water Desalination Using Microbial Desalination Cells. Environ. Sci. Technol. 2009, 43, 7148–7152.
[2] H. Luo, P. Xu, T. Roane and Z. Ren, Microbial desalination cells for improved performance in wastewater treatment, electricity production, and desalinationBioresour. Technol., 2012, 105,60–66.
[3]P. Xu, J. E. Drewes and D. Heil, Desalination, 2008, 225, 139–155.
[4] Casey Forrestal,a Pei Xub and Zhiyong Ren. Sustainable desalination using a microbial capacitive desalination cell. Energy Environ. Sci., 2012, 5, 7161
[5]. Casey Forrestal,a Zachary Stoll,b Pei Xu*b and Zhiyong Jason Ren. Microbial capacitive desalination for integrated organic matter and salt removal and energy production from unconventional natural gas produced water. Environ. Sci.: Water Res. Technol., 2015, 1, 47
[6] L. F. Greenlee, D. F. Lawler, B. D. Freeman, B. Marrot and P. Moulin, Water Res., 2009, 43, 2317–2348
[7] K. L. Hickenbottom, N. T. Hancock, N. R. Hutchings, E. W. Appleton, E. G. Beaudry, P. Xu and T. Y. Cath, Desalination, 2013, 312, 60–66.
[8] J. E. Drewes, N. T. Hancock, K. L. Benko, K. Dahm, P. Xu, D. Heil and T. Y. Cath, Treatment of coalbed methane producedwater. 126–128, Colorado School of Mines, Golden, CO., 2009.
[9] S. Mondal and S. R. Wickramasinghe, J. Membr. Sci., 2008, 322, 162–170.
[10] M.I. Kerwick, S.M. Reddy, A.H.L. Chamberlain, D.M. Holt, Electrochim. Acta 50 (2005) 5270.
[11] S. Porada, R. Zhao, A. Van Der Wal, V. Presser, P. Biesheuvel, Review on the science and technology of water desalination by capacitive deionization, Progress in Materials Science, 58 (2013) 1388-1442.
[12] S. Porada,ab D. Weingarth,b H. V. M. Hamelers,a M. Bryjak,c V. Presser and P. M. Biesheuvel. Carbon flow electrodes for continuous operation of capacitive deionization and capacitive mixing energy generation. J. Mater. Chem. A, 2014, 2, 9313.